The freeze fracture technique has emerged as a groundbreaking method in the realm of cell biology, offering unprecedented insights into the architecture of cellular membranes. This technique, introduced in the mid-20th century, has revolutionized our understanding of the intricate details of cell membranes, which are pivotal to numerous biological processes. By enabling researchers to examine the interior of membranes in their natural state, the freeze fracture technique has provided a window into the complex world of cellular structures, revealing features that were once elusive to scientists.
At its core, the freeze fracture technique involves rapidly freezing biological specimens and then fracturing them to reveal the interior surfaces of cellular membranes. This process is followed by a shadowing with metals to create a replica that can be observed under an electron microscope. The resulting images offer a detailed view of the membrane's inner landscape, including the distribution and organization of proteins and lipids. This has been particularly useful in studying various cellular processes, such as signal transduction, ion transport, and membrane fusion, all of which are critical for maintaining cellular function and homeostasis.
As we delve deeper into the intricacies of the freeze fracture technique, it becomes evident that this method has not only enhanced our understanding of cellular membranes but also paved the way for future innovations in cell biology and medicine. By providing a clearer picture of membrane architecture, this technique has facilitated the development of targeted therapies and interventions aimed at addressing a wide array of diseases. In this comprehensive article, we will explore the history, methodology, applications, and future prospects of the freeze fracture technique, shedding light on its pivotal role in advancing the field of biological sciences.
Table of Contents
- A Glimpse into the History of Freeze Fracture Technique
- Principles and Methodology of Freeze Fracture
- Preparation of Specimens for Freeze Fracture
- The Fracturing Process
- Replication and Shadowing
- Role of Electron Microscopy in Freeze Fracture
- Applications in Cell Biology and Medicine
- Advantages and Limitations of Freeze Fracture Technique
- Comparative Analysis with Other Techniques
- Recent Innovations and Improvements
- Case Studies Demonstrating Impact
- Future Prospects and Research Directions
- Frequently Asked Questions
- Conclusion
A Glimpse into the History of Freeze Fracture Technique
The origins of the freeze fracture technique can be traced back to the mid-20th century, a period marked by rapid advancements in electron microscopy and cellular biology. The technique was pioneered by scientists who sought to overcome the limitations of traditional microscopy methods, which often failed to reveal the detailed structure of cellular membranes. This quest for a more detailed understanding of cell membranes led to the development of the freeze fracture technique, which has since become a cornerstone of cell biology.
Initially, the technique was met with skepticism due to its complexity and the challenges associated with interpreting the resulting images. However, as researchers refined the method and gained a deeper understanding of its potential, the freeze fracture technique quickly gained traction in the scientific community. The ability to visualize the interior surfaces of membranes provided a wealth of new information about the organization and function of membrane proteins and lipids, which are essential components of cellular processes.
Over the years, the freeze fracture technique has undergone numerous refinements and adaptations, allowing it to be applied to a wide range of biological specimens. This has expanded its utility beyond basic research, enabling its application in clinical and biomedical research. As we look back at the history of the freeze fracture technique, it is clear that this method has played a pivotal role in advancing our understanding of cellular membranes and their functions.
Principles and Methodology of Freeze Fracture
The freeze fracture technique is based on a series of carefully orchestrated steps that allow researchers to visualize the interior surfaces of cellular membranes. The process begins with the rapid freezing of biological specimens, which is achieved using liquid nitrogen or other cryogenic substances. This rapid freezing is crucial as it preserves the native state of the membranes, preventing the formation of ice crystals that can damage the delicate structures.
Once frozen, the specimen undergoes fracturing, a process that involves splitting the specimen along natural lines of weakness. In the case of cellular membranes, these lines typically occur between the lipid bilayers, revealing the inner surfaces of the membrane. This is followed by a process known as shadowing, where the fractured surfaces are coated with a thin layer of metal, such as platinum or gold, to create a replica of the surface. This metal replica is then stabilized with a carbon layer and observed under an electron microscope to produce detailed images of the membrane's architecture.
The methodology of freeze fracture is both an art and a science, requiring precision and expertise to achieve the best results. Each step in the process is critical, from the initial freezing to the final imaging, and any deviations can affect the quality of the resulting images. Despite these challenges, the freeze fracture technique remains a powerful tool for studying the structural intricacies of cellular membranes.
Preparation of Specimens for Freeze Fracture
The preparation of specimens for freeze fracture is a meticulous process that requires careful handling and precise conditions to preserve the integrity of the cellular structures. The first step involves selecting the appropriate biological specimen, which can range from whole cells to tissue samples, depending on the research objective. Once selected, the specimen is typically fixed using chemical fixatives to stabilize the cellular components and prevent degradation during the freezing process.
Following fixation, the specimen is cryoprotected using solutions such as glycerol or sucrose, which help prevent the formation of ice crystals during freezing. This step is essential for preserving the native state of the membranes and ensuring that the delicate structures are not disrupted. Once cryoprotected, the specimen is rapidly frozen, often by plunging it into liquid nitrogen or using a high-pressure freezing device. This rapid freezing is crucial for maintaining the structural integrity of the membranes and preventing ice crystal formation.
The preparation of specimens for freeze fracture requires a combination of technical skill and attention to detail, as even minor deviations can affect the quality of the resulting images. By ensuring that each step is carefully executed, researchers can obtain high-resolution images that provide valuable insights into the architecture of cellular membranes.
The Fracturing Process
The fracturing process is a critical step in the freeze fracture technique, as it involves splitting the frozen specimen to reveal the interior surfaces of cellular membranes. This process is typically carried out in a vacuum chamber at cryogenic temperatures to prevent sublimation and ensure that the specimen remains in its frozen state. The fracturing is achieved using a microtome or a specially designed knife, which carefully cleaves the specimen along natural lines of weakness.
In the case of cellular membranes, these lines of weakness often occur between the lipid bilayers, allowing researchers to access the membrane's inner surfaces. The resulting fracture planes provide a unique perspective of the membrane's architecture, revealing details such as the distribution and arrangement of proteins and lipids. This information is invaluable for understanding the functional properties of membranes and their role in various cellular processes.
The fracturing process requires precision and expertise, as any errors can result in damage to the specimen or loss of structural information. By carefully controlling the conditions and using specialized equipment, researchers can achieve high-quality fractures that provide detailed insights into the complex world of cellular membranes.
Replication and Shadowing
Following the fracturing process, the next step in the freeze fracture technique is replication and shadowing, which involves creating a metal replica of the fractured surface. This is achieved by coating the surface with a thin layer of metal, such as platinum or gold, which is applied at an angle to create shadows and enhance the three-dimensional appearance of the structures. This process is known as shadowing, and it is essential for providing contrast in the resulting images.
Once shadowed, the replica is stabilized with a carbon layer, which provides additional support and prevents deformation. The metal-carbon replica is then carefully separated from the underlying biological material, leaving behind a detailed representation of the fractured surface. This replica can be observed under an electron microscope, allowing researchers to obtain high-resolution images that reveal the intricate details of the membrane's architecture.
Replication and shadowing are critical steps in the freeze fracture technique, as they determine the quality and accuracy of the resulting images. By carefully controlling the conditions and using precise techniques, researchers can obtain replicas that provide valuable insights into the structure and function of cellular membranes.
Role of Electron Microscopy in Freeze Fracture
Electron microscopy plays a pivotal role in the freeze fracture technique, as it allows researchers to visualize the metal replicas of fractured surfaces at high resolution. Unlike traditional light microscopy, electron microscopy uses a beam of electrons to create detailed images of the specimen, providing unparalleled insights into the architecture of cellular membranes.
The electron microscope used in freeze fracture is typically a transmission electron microscope (TEM), which allows for the observation of thin sections of the replica. By passing a beam of electrons through the replica, the TEM creates detailed images that reveal the distribution and arrangement of proteins and lipids within the membrane. These images provide valuable information about the structural organization of membranes and their role in various cellular processes.
The use of electron microscopy in freeze fracture has been instrumental in advancing our understanding of cellular membranes, providing insights that were previously inaccessible using traditional microscopy techniques. By harnessing the power of electron microscopy, researchers can obtain high-resolution images that offer a deeper understanding of the complex world of cellular structures.
Applications in Cell Biology and Medicine
The freeze fracture technique has found numerous applications in cell biology and medicine, providing valuable insights into the structure and function of cellular membranes. In cell biology, the technique has been used to study a wide range of cellular processes, including signal transduction, ion transport, and membrane fusion. By revealing the distribution and arrangement of proteins and lipids within the membrane, freeze fracture has provided a deeper understanding of the mechanisms underlying these processes.
In medicine, the freeze fracture technique has been used to study the structural abnormalities associated with various diseases, such as cancer, neurodegenerative disorders, and infectious diseases. By providing a detailed view of the membrane's architecture, the technique has facilitated the development of targeted therapies and interventions aimed at addressing these diseases. Additionally, freeze fracture has been used to study the effects of drugs on cellular membranes, providing valuable information for drug development and optimization.
The applications of freeze fracture in cell biology and medicine are vast and continue to expand as researchers uncover new insights into the complex world of cellular membranes. By providing a detailed view of the membrane's architecture, the technique has paved the way for future innovations in the field of biological sciences.
Advantages and Limitations of Freeze Fracture Technique
The freeze fracture technique offers several advantages that make it a powerful tool for studying cellular membranes. One of the key advantages is its ability to provide high-resolution images of the membrane's interior surfaces, revealing details that are often inaccessible using traditional microscopy techniques. This level of detail is invaluable for understanding the structural organization of membranes and their role in various cellular processes.
Another advantage of the freeze fracture technique is its ability to preserve the native state of the membranes, allowing researchers to study the structures in their natural environment. This is achieved through the rapid freezing process, which prevents the formation of ice crystals and preserves the delicate structures. Additionally, the technique is highly versatile and can be applied to a wide range of biological specimens, from whole cells to tissue samples.
Despite these advantages, the freeze fracture technique also has some limitations. One of the main challenges is the complexity of the process, which requires precision and expertise to achieve high-quality results. Additionally, the interpretation of the resulting images can be challenging, as the technique provides only a two-dimensional view of the three-dimensional structures. This can make it difficult to fully understand the arrangement and function of the membrane components.
Despite these limitations, the freeze fracture technique remains a valuable tool for studying cellular membranes, providing insights that are essential for advancing our understanding of biological processes.
Comparative Analysis with Other Techniques
The freeze fracture technique is one of several methods used to study the structure of cellular membranes, each with its own strengths and limitations. One of the most common techniques used in conjunction with freeze fracture is cryo-electron microscopy (cryo-EM), which also involves the rapid freezing of specimens. Cryo-EM offers the advantage of providing three-dimensional reconstructions of the membrane structures, allowing for a more comprehensive understanding of their organization and function.
Another technique often compared to freeze fracture is atomic force microscopy (AFM), which provides high-resolution images of the membrane surface without the need for freezing or fracturing. AFM offers the advantage of being able to study live cells and dynamic processes, providing valuable information about the mechanical properties of the membranes.
While each technique offers unique advantages, the freeze fracture technique remains a valuable tool for studying the detailed architecture of cellular membranes. By combining the strengths of different techniques, researchers can obtain a more comprehensive understanding of the complex world of cellular structures.
Recent Innovations and Improvements
Recent years have seen numerous innovations and improvements in the freeze fracture technique, enhancing its utility and expanding its applications. One of the key advancements has been the development of high-pressure freezing devices, which allow for rapid freezing of specimens without the need for cryoprotectants. This has improved the preservation of the native state of the membranes and reduced the risk of ice crystal formation.
Another significant improvement has been the integration of advanced imaging techniques, such as cryo-electron tomography, which allows for three-dimensional reconstructions of the membrane structures. This has provided a more comprehensive understanding of the arrangement and function of the membrane components, offering new insights into their role in cellular processes.
Additionally, recent advancements in computational analysis have improved the interpretation of the resulting images, allowing researchers to extract more detailed information about the membrane's architecture. By leveraging these innovations, researchers can obtain a deeper understanding of the complex world of cellular membranes, paving the way for future discoveries in cell biology and medicine.
Case Studies Demonstrating Impact
The freeze fracture technique has been instrumental in numerous breakthroughs in cell biology and medicine, as demonstrated by several case studies. One notable example is the study of the structure and function of gap junctions, which are critical for cell-to-cell communication. By using freeze fracture, researchers were able to visualize the arrangement of gap junction proteins, providing valuable insights into their role in signal transduction and cellular communication.
Another case study involved the use of freeze fracture to study the effects of cholesterol on membrane organization. By examining the distribution of lipids and proteins within the membrane, researchers were able to gain a deeper understanding of the role of cholesterol in maintaining membrane fluidity and stability. This information has been critical for understanding the mechanisms underlying various diseases, such as cardiovascular disease and neurodegenerative disorders.
These case studies highlight the significant impact of the freeze fracture technique in advancing our understanding of cellular membranes and their role in various biological processes. By providing detailed insights into the architecture of membranes, the technique has facilitated numerous discoveries and innovations in the field of biological sciences.
Future Prospects and Research Directions
The future of the freeze fracture technique is bright, with numerous opportunities for further advancements and applications. One promising area of research is the integration of freeze fracture with other imaging techniques, such as super-resolution microscopy and cryo-electron tomography, to provide a more comprehensive understanding of membrane structures. By combining the strengths of different techniques, researchers can obtain a more detailed view of the complex world of cellular membranes.
Another potential area of research is the application of freeze fracture to study dynamic processes, such as membrane fusion and fission, in real-time. By developing new methods and technologies, researchers can gain a deeper understanding of the mechanisms underlying these processes, providing valuable insights for the development of targeted therapies and interventions.
As we look to the future, it is clear that the freeze fracture technique will continue to play a critical role in advancing our understanding of cellular membranes and their role in various biological processes. By harnessing the power of this technique, researchers can unlock new insights and pave the way for future discoveries in cell biology and medicine.
Frequently Asked Questions
1. What is the freeze fracture technique used for?
The freeze fracture technique is used to study the structure of cellular membranes by revealing the interior surfaces of membranes, providing detailed insights into the distribution and arrangement of proteins and lipids.
2. How does the freeze fracture technique work?
The technique involves rapidly freezing biological specimens, fracturing them to reveal the interior surfaces, and creating a metal replica that is observed under an electron microscope to produce detailed images of the membrane's architecture.
3. What are the advantages of the freeze fracture technique?
The technique offers high-resolution images of membrane surfaces, preserves the native state of membranes, and can be applied to a wide range of biological specimens, providing valuable insights into the structural organization and function of membranes.
4. What are the limitations of the freeze fracture technique?
The technique is complex and requires precision and expertise, and it provides only a two-dimensional view of three-dimensional structures, which can make interpretation challenging.
5. How does freeze fracture compare to other techniques?
While freeze fracture provides detailed views of membrane interiors, other techniques like cryo-electron microscopy and atomic force microscopy offer complementary insights, such as three-dimensional reconstructions and the ability to study live cells.
6. What are the future prospects for the freeze fracture technique?
The future prospects include integrating freeze fracture with other imaging techniques, studying dynamic processes in real-time, and developing new methods to enhance its utility and applications in cell biology and medicine.
Conclusion
The freeze fracture technique has revolutionized our understanding of cellular membranes, providing detailed insights into their structure and function that were previously inaccessible. By revealing the intricate details of membrane architecture, the technique has facilitated numerous discoveries and innovations in cell biology and medicine. Despite its complexity and limitations, the freeze fracture technique remains a powerful tool for studying the complex world of cellular membranes, offering valuable information that is essential for advancing our understanding of biological processes. As researchers continue to refine and innovate the technique, its impact on the field of biological sciences is set to grow, paving the way for future discoveries and advancements.
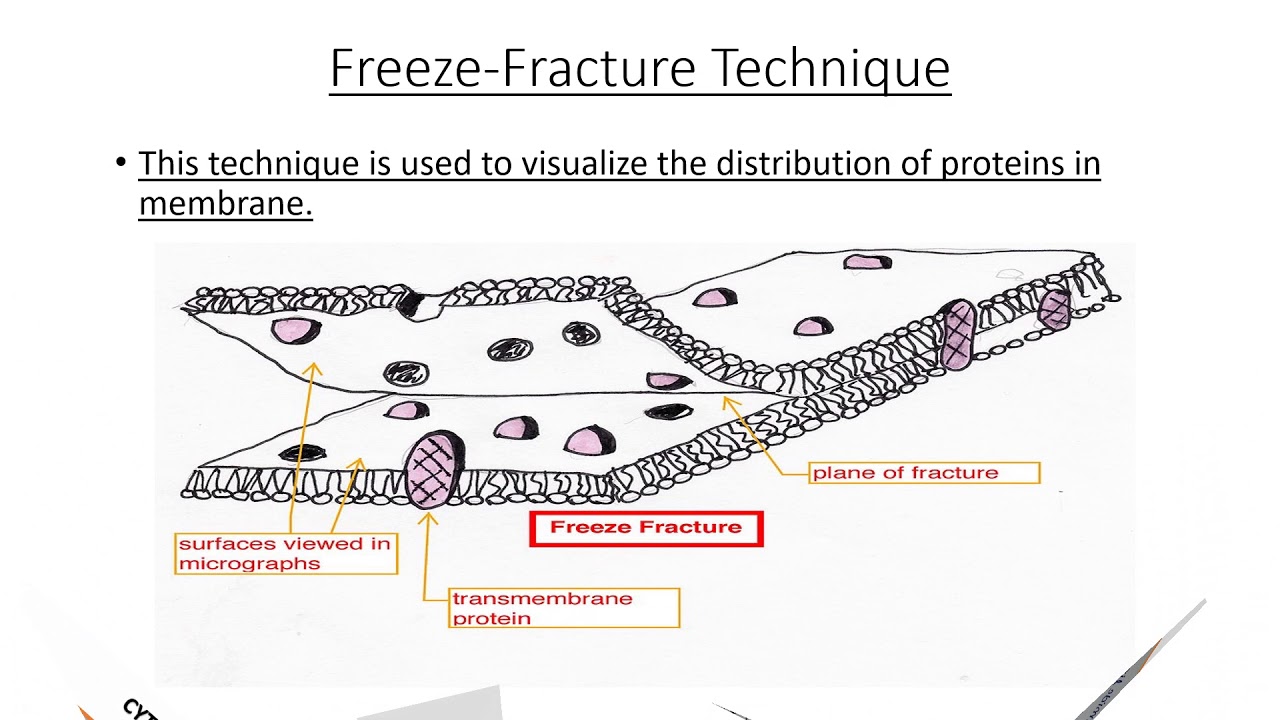
